Thermodynamic signatures of field-induced phase transitions in graphite
In solid-state physics, the free electron gas is a well-known and simple model for the behaviour of valence electrons in a crystal structure of a metal. Valence electrons are completely detached from their ions and form a gas of non-interacting electrons. Here, the crystal lattice is not explicitly taken into account. Given its simplicity, this model is surprisingly successful in explaining many experimental phenomena.
When a magnetic field is applied to the free electron gas, their energy states are quantized into the so-called Landau levels. This means, the charged particles can only occupy orbits with discrete energy values. As the magnetic field increases, there are fewer Landau levels occupied, until finally all the carriers are confined in the lowest Landau level.
The studied material Graphite is a macroscopic stack of graphene layer. In each graphene layer, carbon atoms are arranged in a honeycomb lattice. This semimetal Graphite is a model system for the study of electrons in the so-called magnetic quantum limit. This quantum limit is attained when the magnetic field is strong enough to confine the carriers to their lowest Landau levels. With available magnetic fields, it is only accessible in solids with charge carrier concentration as low as in Graphite. Here, above the quantum limit of 7.5 T, the electrons are restricted to the lowest Landau level. In contrast, for a normal metal like copper, the quantum limit would be reached with a magnetic field as high as several thousand Tesla.
It is expected that the model of the free electron gas failures beyond this limit since here electron interactions may play a significant role in determining the electronic ground state. Indeed, in the early 1980s, a phase transition induced by a magnetic field above 20 T was discovered in Graphite by magneto-resistance. Recent transport measurements up to 80 T revealed a second field-induced instability. Such phase transitions can only occur due to electron-electron interaction. Up to now, the nature of these anomalies was still unknown.
In our work, in an international collaboration instigated by Kamran Behnia and Benoît Fauqué from Paris, we have studied Graphite at the High Field Magnet Laboratory (HFML) in Nijmegen and at the Laboratoire National des Champs Magnetiques Intenses in Grenoble/Toulouse applying ultrausound, magnetostriction and electrical transport measurements.
In our studies, we found a rich phase diagram characterized not only by two transitions but by a sequence of several thermodynamic phase transitions. Most important, Ultrasound measurements proved the very first time the thermodynamic nature of these transitions. Our analysis also revealed that the electron-lattice interaction has to be taken into account in theoretical models in addition to electron-electron interaction.
Our experimental contribution to this work was mainly to study the thermodynamic signatures with magnetostriction measurements. The weakness of these transitions and the limited space of the used cryogenic devices has made this a particular complicated task. For this reason, Robert Küchler has developed the world’s smallest high-resolution capacitive dilatometer (see also: Rev. Sci. Instrum. 88, 083903 (2017)). Meanwhile he is also marketing the precision instrument in a spin-off company http://www.dilatometer.info/. The new super compact design enables now to measure magnetostriction up to 37.5 T in a bitter magnet at HFML in Nijmegen. With the commissioning of the new hybrid magnet in 2018, the field range will be expanded even further, to 45 T.
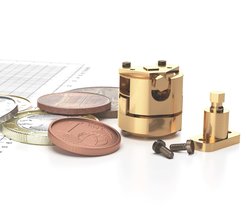
Fig.1) The world’s smallest high-resolution capacitive dilatometer: A sample is clamped between the two plates of a capacitor whose capacitance changes when the sample expands or contracts. This change in capacitance can be measured very accurately. The dilatometer enables to measure changes in length of ΔL ≈ 10-11 - 10-12 m which are about hundred times smaller than the separations of the atoms in the crystal lattice. The scientific instrument can be operated from room temperature down to 10 mK.
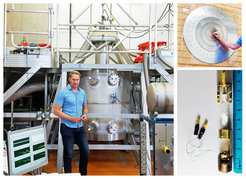
Fig.2) Left: picture of the water-cooled, resistive Bitter magnet at the HFML in Nijmegen. The magnet can reach a static field of 37.5 T and is cooled by a huge water flow of 10 000 l/min. In spite of the hereby-caused vibrational noise we could resolve changes in length of ΔL ≈ 10-11 - 10-10 m. Top right: A view down of the huge magnet coils and the very limited space in the middle. Bottom right: mini-dilatometer mounted on a very large probe (2 m). The ruler measures cm. For more details, see Rev. Sci. Instrum. 88, 083903 (2017).