Theory of Topological Quantum Systems -
X. Feng
Topological materials have fascinating properties and are a success of theoretical material science since their existence was first theoretically predicted in 2006 [1,2] before they were experimentally discovered [3]. Since, they have dominated the landscape of solid-state physics owing to their potential applications. Topological insulators (TIs) exhibit a gapped bulk and a gapless surface, wherein electron conduction occurs perfectly, because the electrons are topologically protected from back scattering in the presence of crystalline symmetries and/or time-reversal symmetry (TRS) [4]. This unique property makes topological materials potential candidates for several applications, such as more energy-efficient microelectronic components, better catalysts, improved thermoelectric converters, and new magnetic storage media. For the first 10 years, the barrier between topological properties and applications was the inability to identify an optimal material. In 2017, the principal investigator (PI) and collaborators presented a new formalism called Topological Quantum Chemistry (TQC), which provides a description of the universal global properties of all possible bands in weakly interacting materials [5] and facilitates efficient topological materials search. In 2019, we developed a code based on TQC [6] to perform a high throughput search of materials in a given database.
The findings changed our understanding of the number of naturally occuring topological materials. Approximately 40%−50% of all non-magnetic materials can be classified as topological at the Fermi level, which gives us a ‘periodic table’ of topological materials. We have uploaded our topological materials catalogue to a public website www.topologicalquantumchsmitry.com (see Fig. 1). However, the outcomes of TQC and the high throughput surpass the discovery of new materials; these help us discover new topological phases such as high-order topological materials [7,8] or the intriguing fragile phase [9] (see Fig. 2) detected in the twisted bilayer graphene [10].
![Fig. 2: Band structure and Wilson loops of a fragile phase in a honeycomb lattice. Figure taken from [9].](/3320922/original-1624447781.jpg?t=eyJ3aWR0aCI6MjQ2LCJvYmpfaWQiOjMzMjA5MjJ9--0a567b2d09a849ab9b8b796da4e8ede9275e4f7e)
Fig. 2: Band structure and Wilson loops of a fragile phase in a honeycomb lattice. Figure taken from [9].
Recently, we extended our TQC formalism to magnetic materials, obtaining new high throughputs in the magnetic database of the Bilbao Crystallographic Server, and discovered ~100 new topological magnetic materials [11]; details of these have also been uploaded to the TQC website.
Many of the unique and outstanding phenomena observed in condensed matter physics are attributed to electron–electron interactions, and ab initio codes only consider very weak ones, and thus, further research is required to achieve materials realisation using the TQC formalism presented. In this project, we plan to bridge the knowledge gap between first-principles based materials and the realisation of materials in devices, with the electronic bands structure as a solid starting point. Furthermore, in addition to strong topology, interesting surface states can be identified in so-called obstructed atomic limit (OAL) materials. In these materials, the electronic charge is displaced from the atomic charge, and a robust surface state can be identified for certain terminations.
We also plan to develop new theoretical and numerical frameworks for studying the electronic and magnetic properties of OAL, developing sophisticated pseudopotentials so that TCQ can be applied to correlated materials. We will also investigate the influence of correlations on electronic properties and predict measurable universal features. These developments will allow us to study realistic materials and systems and understand their topological properties and applications. In particular, we will focus on oxides because they are chemically robust and have diverse applications. Fig. (3) shows the electronic band structure of a prototype OAL material.
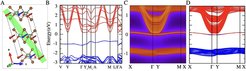
Fig 3: Band structure of an OAL oxide. (A) Crystal structure. (B) Bulk band structure. (C) ARPES simulation of the surface state. (D) Band structure calculations of the (001) surface