Ultra-high precision thermodynamics of unconventional superconductivity
It is always exciting to be able to use a new experimental technique to uncover new physics. This is just what a team led from our Institute, with collaborators from Karlsruhe, St Andrews, Cornell, Tsukuba and Stanford report in a paper published today [1]. Working with a technique called the ac elastocaloric effect, we have mapped out the so-called phase diagram of the unconventional superconductor Sr2RuO4.
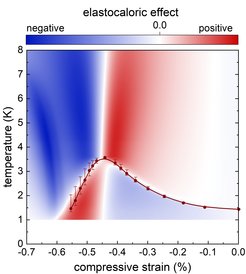
The figure has been adopted from Ref. [1], licensed under a BY-CC 4.0 License, http://creativecommons.org/licenses/by/4.0/
In the elastocaloric effect, thermodynamics tells us that the temperature of a crystal must change if its properties change when it is subjected to uniaxial pressure, i.e. squeezed along one particular direction. The problem is that the expected changes are very small. Over the past decade, one of the main goals of our group has been the development of methods in which the pressure is applied by voltage-controlled actuators known as piezoelectric stacks. The ac elastocaloric effect makes use of the possibility to add a tiny ac voltage to the dc voltage that is used to apply the bulk pressure, adding a tiny oscillatory pressure [2]. This results in a tiny temperature oscillation of the sample. In our experimental conditions, the oscillatory signal is approximately one thousandth of a degree kelvin when the sample is at 8 degrees. By working with ac techniques and special low temperature amplification, we have a signal-to-noise ratio of 500 even of this tiny signal, producing extremely high-resolution data.
We show how the unusual superconductivity depends on pressure, and how it relates to a magnetic phase at high pressures, demonstrating a previously unappreciated features that relate Sr2RuO4 to other unconventional superconductors. Using results from separate ultrasound velocity measurements performed at Cornell, we calibrate our signal in absolute units. This allows us to compare experiment and a theory specially developed for this project, and to calculate one of the most important properties of any material, its entropy, as a function of temperature and pressure.
One of the really fun aspects of the project was that every aspect of our signal was new, so unravelling what was going on was an ongoing challenge. Understanding it was a real team effort between experimental and theoretical physics. Our results both narrow down the on-going, twenty-five-year quest to understand the superconductivity of Sr2RuO4 and set a benchmark for future work on a host of other systems.
[1] Y.-S. Li, M. Garst, J. Schmalian, S. Ghosh, N. Kikugawa, D.A. Sokolov, C.W. Hicks, F. Jerzembeck, M.S. Ikeda, Z. Hu, B. Ramshaw, A.W. Rost, M. Nicklas & A.P. Mackenzie Nature 607, 276–280 (2022).
[2] M.S. Ikeda, J.A.W. Straquadine, A.T. Hristov, T. Worasaran, J.C. Palmstrom, M. Sorensen, P. Walmsley, & I.R. Fisher, Rev. Sci. Inst. 90, 083902 (2019).